Repetitive Transcranial Magnetic Stimulation (rTMS) in Depression: Protocols, Mechanisms and New Developments
Arns, M.1,2,3, Iseger, T.1,3, Spronk, D. B. 1, Brown, T.4& Fitzgerald, P. B.5
- 1 Research Institute Brainclinics, Nijmegen, The Netherlands
- 2 neuroCare Group, Munich, Germany
- 3 Dept. of Experimental Psychology, Utrecht University, Utrecht, The Netherlands
- 4 neuroCare Group, Melbourne, Australia
- 5 Epworth Healthcare and Monash Alfred Psychiatry Research Centre, Melbourne, Australia
Updated and revised version of: Repetitive transcranial magnetic stimulation in depression: Protocols, mechanisms and new developments. In Neuromodulation and neurofeedback: Techniques and applications. Elsevier.
Abstract
This book chapter introduces and explains protocols, mechanisms and new developments of transcranial magnetic stimulation (TMS) in the treatment of depression. Repetitive TMS protocols for depression can mainly be differentiated based on the stimulation location and frequency, but trains, sessions and inter train intervals are also parameters that can be manipulated. Various neuroimaging findings that shed light on potential antidepressant mechanisms are discussed, including the role of the depression network, network connectivity and optimizing rTMS using such knowledge. Furthermore, new developments in the field of engineering, in particular the development of the H-coil, and new protocols are reviewed. The chapter finishes with a discussion of these new insights and potential implementations that could improve and enhance treatment response to rTMS.
Introduction
TMS (transcranial magnetic stimulation) is a non-invasive neuromodulation technique. It has a very direct influence on brain physiology. The basic principle of TMS is the application of short magnetic pulses over the scalp of a subject with the aim of inducing electrical currents in the neurons of the cortex. A typical TMS device consists of a stimulator that can generate a strong electrical current, and a coil in which the fluctuating electrical current generates magnetic pulses. If the magnetic pulses are delivered in the proximity of a conductive medium, e.g. the brain, a secondary current in the conductive material (e.g. neurons) is induced (Figure 1). In the practice of TMS, a subject is seated in a chair and an operator positions the coil above the scalp of the subject, and the TMS pulses are applied.
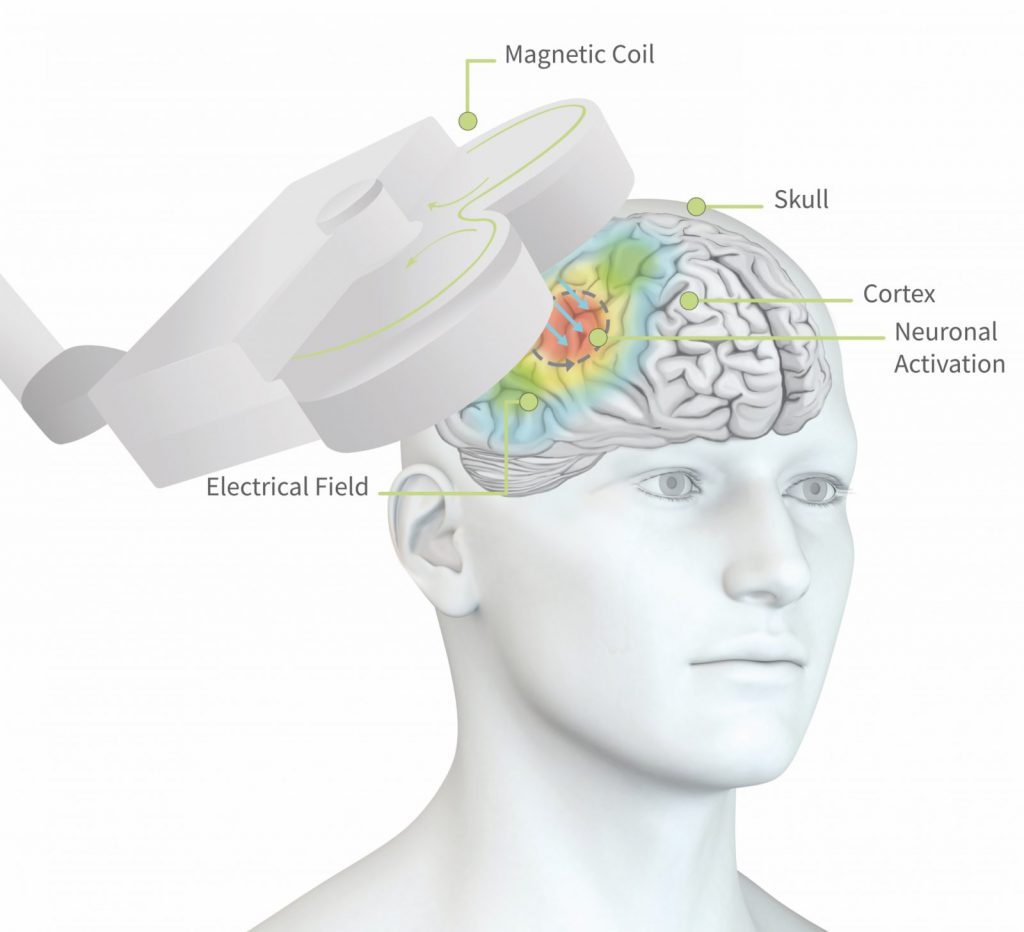
Rtms25 S
Figure 1: Visual illustration of the induction of electrical currents in the brain (grey arrows in the brain) through the magnetic pulses applied by means of the coil (grey 8-shaped figure) positioned above the head. The colors on the scalp reflect the electrical field, i.e. where neural activation is most focused. Figure used with permission from neuroCare Group ©.
Anthony Barker and his colleagues at the University of Sheffield were the first to develop a TMS device, introducing a new neuromodulation technique in neuroscience. The new application, demonstrated first by these researchers, was the induction of a motor evoked potential (e.g. activating the muscles abducting the thumb) by means of applying a TMS pulse over the primary motor cortex (Barker, Jalinous, & Freeston, 1985).
Initially, TMS was used mainly in studies on motor conductivity through investigating the temporal aspects and amplitude of the evoked motor responses after stimulating the motor cortex. Continuing progress on the technical aspects of TMS devices soon made it possible to deliver multiple pulses within a short time period, i.e. repetitive TMS (rTMS). With the development of rTMS, researchers were able to induce changes that outlasted the stimulation period (Pascual-Leone et al., 1999). This has led to a considerable extension of the possible applications of TMS. Currently, rTMS is used for an increasing variety of applications such as the study of pathophysiology of diseases, the investigation of the contribution of certain brain regions to particular cognitive functions and, most relevant for this chapter, the treatment of psychiatric diseases – most specifically, depression.
The potential of repetitive TMS in the treatment of psychiatric disorders was suggested for the first time relatively soon after the development of the first TMS device in 1985. In a study on motor conductivity, changes in mood in several healthy volunteers who received single pulses over the motor cortex were described (Bickford, Guidi, Fortesque, & Swenson, 1987). Following this initial observation, the technical progress and the increasing availability of TMS devices has led to the further investigation of rTMS in the treatment of depression. Apart from being the first investigated psychiatric application, it is also the most well investigated psychiatric application. Major depression has a disabling effect on daily activity, indicating that effective treatment is crucial. Treatment with antidepressant medication is the most common and first line treatment for many individuals. However, a significant percentage of patients are treatment resistant (TRD) meaning that they do not sufficiently respond to antidepressant medication (Keller et al., 2000; Kirsch et al., 2008; Rush et al., 2006). Some of these patients proceed to electroconvulsive therapy (ECT). Despite remarkable clinical results (Husain et al., 2004), ECT is a controversial and unpopular treatment option due to the required induction of a seizure and associated side-effects such as memory complaints (Robertson and Pryor, 2006).
Following initial positive results with depression, and due to its painless and non-invasive administration, rTMS has been proposed as a ‘better’ alternative to ECT (Paus & Barrett, 2004) or as an alternative for patients who may not be willing to undergo ECT, or for whom ECT may not be suitable. In order to compare efficacy of these treatments, rTMS and ECT have been jointly investigated in several studies (Eranti et al., 2007; Rosa et al., 2006). Of the several studies performed Eranti et al. (2007) observed a great advantage for ECT. However, others (Grunhaus, Schreiber, Dolberg, Polak, & Dannon, 2003; Pridmore, Bruno, Turnier-Shea, Reid, & Rybak, 2000; Rosa et al., 2006) found comparable efficacy rates for ECT and rTMS in the treatment of depression. Furthermore, Eranti et al. (Eranti et al., 2007) included patients with psychotic depression whereas the other studies only involved non-psychotic depression (Pridmore et al., 2000), suggesting that rTMS may not be indicated for the treatment of depression with psychotic features. This notion was further supported by a meta-analysis from Berlim and colleagues (Berlim, Van den Eynde, & Daskalakis, 2013) comparing ECT and rTMS studies in the treatment of depression. They found an overall effect size of 1.42 favoring ECT, which dropped to a small effect size of 0.3 when studies that included psychotic depression were excluded.
The early reports of rTMS as an antidepressant treatment modality consisted of pilot studies with small numbers of subjects. In these early studies arbitrary stimulation parameters over various and non-specific brain regions were applied (Hoflich G, Kasper S, Hufnagel A, Ruhrmann S, Moller HJ 1993). A report by George and colleagues (George et al., 1995) showed robust improvements in depressive symptoms in two out of six patients. This study marked the start of the serious pursuit of rTMS as a potential treatment option for depressed patients. Subsequently, a reasonably large number of open label as well as randomized sham-controlled studies were performed. Most studies found a moderately favorable treatment effect for rTMS using various designs (Avery et al., 2006; Fitzgerald et al., 2006, 2003; Garcia-Toro et al., 2001; Mogg et al., 2008; O’Reardon et al., 2007; Padberg et al., 1999; Rossini, Lucca, Zanardi, Magri, & Smeraldi, 2005), which has recently been confirmed by several meta-analyses (Schutter 2009, 2010), two independent multicenter randomized controlled trials (George et al., 2010; O’Reardon et al., 2007) as well as a level A evidence based on the recently published rTMS guidelines (Lefaucheur et al., 2014).
After more than 20 years of research, rTMS is increasingly considered an acceptable intervention for treatment resistant depression (TRD). Most notably, the above-mentioned two independent multicenter randomized controlled studies (George et al., 2010; O’Reardon et al., 2007) and rTMS guidelines (Lefaucheur et al., 2014) have been instrumental in this process. rTMS is currently approved by the FDA in the United States for TRD, both for ‘regular rTMS’ as well as ‘deep-rTMS’ (discussed below). In addition, a growing number of private outpatient as well as hospitalized depression patients are being treated in clinical settings worldwide. Repetitive TMS treatment for depression is now being reimbursed by insurance companies in more and more countries e.g. the US and the Netherlands.
In this chapter, a comprehensive overview of rTMS in the treatment of depression will be provided. In the first section various rTMS protocols will be reviewed in terms of the different stimulation parameters of interest. Subsequently, potential neural mechanisms associated with antidepressant outcomes will be reviewed. Finally, new developments in the field are addressed.
Protocols
The clinical and physiological effects of rTMS have been found to depend on the frequency, intensity and duration of stimulation (e.g. O’Reardon et al., 2007; Avery et al., 2006; Fitzgerald et al., 2006b; Padberg et al., 2002). The most important distinguishing parameters for rTMS protocols in depression are the stimulation frequency and the stimulation location. These will be discussed at length by reviewing literature that used diverse choices for these parameters. Some other relevant parameters (intensity, number of trains, inter train interval and number of sessions) will be briefly described. In Figure 2, some of the characteristics of an rTMS stimulation protocol are illustrated.
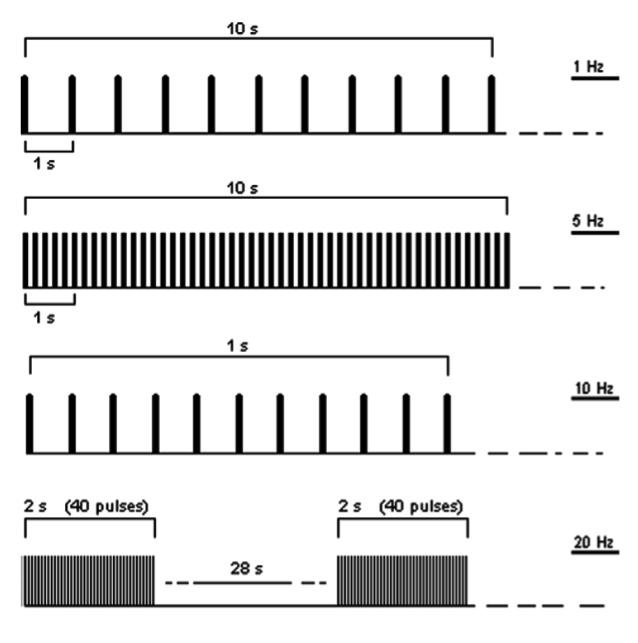
Rtms Figure2
Figure 2: Examples of 10 s of rTMS at 1 Hz (first trace) and at 5 Hz (second trace); 1 s of rTMS at 10 Hz and an example of 20 Hz application (trains of 2 s interleaved by a pause of 28 s). Figure taken and adapted from Rossi et al. (Rossi, Hallett, Rossini, Pascual-Leone, & The Safety of TMS Consensus Group, 2009).
Progress in the development of technical aspects of TMS devices and advancing insights have led to a continuing progression of experimental and innovative protocols. Some more recently developed protocols investigated in the treatment of depression, such as theta burst stimulation and deep TMS stimulation, are discussed in the section ‘new developments’.
Stimulation frequency
The stimulation frequency refers to the number of pulses delivered per second, as can be programmed on the TMS device. Examination of stimulation frequencies in rTMS studies in depression reveals that, at first glance, two types of studies can be discerned: studies performing high frequency (also referred to as fast) rTMS (HF-rTMS) and studies in which low frequency (also referred to as slow) rTMS (LF-rTMS) parameters are applied. HF-rTMS usually includes frequency parameters of 5Hz or above, whilst LF-rTMS incorporates stimulation frequencies of 1Hz or below. HF-rTMS is usually applied over the left dorsolateral prefrontal cortex (DLPFC), whilst LF-rTMS is mostly applied over the right dorsolateral prefrontal cortex (see ‘stimulation location’ for a more elaborate overview). In addition to studies applying solely HF-rTMS or LF-rTMS, combined approaches have also been proposed and investigated.
High frequency rTMS.
Most rTMS studies in depression have applied a high-frequency stimulation protocol (Avery et al., 2006; O’Reardon et al., 2007). To date, HF-rTMS protocols have mostly used stimulation frequencies of 10 Hz (but this has varied from 5 to 20 Hz). The largest study (O’Reardon et al., 2007) reported significantly better clinical results in an active rTMS group in comparison to the sham group, as measured by the Hamilton Rating Scale for Depression (HAM-D) scale and the Montgomery Asberg Depression Rating Scale (MADRS). This was a randomized study in which 301 medication-free patients were treated with 10 Hz stimulation frequency. In a non-industry sponsored trial by George and colleagues (2010) these results were independently replicated in a sample of 190 patients. Apart from these large multi-center studies, numerous single site studies applying stimulation frequencies of 10 Hz have been performed. These have shown response rates (defined as more than 50% decrease on the depression scale) between 30-50% (Avery et al. 2006; Garcia-Toro et al., 2001; Mogg et al., 2008; O’Reardon et al., 2007; Padberg et al., 1999; Rossini et al., 2005; George et al., 2010). Most of these studies have been performed in treatment resistant patients. In a study by Fitzgerald and colleagues (Fitzgerald et al., 2006), patients who did not respond to a protocol with frequencies of 1 or 2 Hz (LF-rTMS) were assigned to either a 5Hz or 10Hz HF-rTMS protocol. No significant differences in response to a 5 or 10 Hz protocol were shown. Due to the limited number of studies no definitive conclusions can be drawn, but results suggest that in general HF-rTMS (including 5, 17 or 20 Hz stimulation frequencies) do have an antidepressant effect. However, some reports have shown differential effects of various stimulation parameters, including a report of 9 Hz rTMS tending to be less beneficial than 10 Hz (Arns 2010). To summarize, the most optimal stimulation frequency is not yet known, but 10 Hz rTMS has been most frequently investigated and is therefore most commonly used in clinical settings.
Low frequency rTMS.
In addition to the HF-rTMS studies, several LF-rTMS studies have been performed (Fitzgerald et al., 2003; Januel et al., 2006; Klein et al., 1999). For example, in a large sham-controlled study Klein et al. (Klein et al., 1999) showed that 1 Hz rTMS, in which 70 patients were randomly assigned to sham or active treatment, showed significantly larger improvement in depression scores in the active as compared to the sham group. In the largest controlled study on LF-rTMS in depression, 130 patients were initially assigned to a stimulation protocol of either 1 or 2 Hz (Fitzgerald et al., 2006). Of the 130 patients enrolled, approximately 51% could be classified as responders after 10 days of treatment. Interestingly, the response rates between the 1 Hz and 2 Hz did not significantly differ. Although LF-rTMS is a more recently developed protocol and is less well studied, it appears to have beneficial effects comparable to those of HF-rTMS.
In order to systematically investigate if HF or LF-rTMS is more beneficial, protocols were directly compared (Fitzgerald et al., 2003; Fitzgerald, Hoy, Daskalakis, & Kulkarni, 2009; Isenberg et al., 2005). In a double-blind, randomized, sham-controlled study, 60 treatment resistant patients were divided into three groups; one received HF-rTMS trains to the left prefrontal cortex at 10 Hz, the second group received five LF-rTMS trains at 1 Hz to the right prefrontal cortex and the third group received sham treatment. The clinical results showed that the groups treated with HF-rTMS and LF-rTMS had a similar reduction in depressive symptoms and, for both groups, treatment response was better than within the sham group (Fitzgerald et al., 2003). In another study with a similar aim, 27 subjects were assigned to either HF-rTMS (10Hz) or LF-rTMS (1Hz) rTMS. It was concluded that both treatment modalities appeared to be equally efficacious (Fitzgerald et al., 2009) also supported by a large open-label study (Donse, Padberg, Sack, Rush, & Arns, 2018). Schutter (Schutter 2010), based on a meta-analysis of all randomized controlled LF-rTMS studies in depression, suggested that LF-rTMS might even be more beneficial than HF-rTMS. However, direct comparisons of the effect sizes of HF and LF-rTMS did not show a statistically significant difference due to overlapping confidence intervals. More research with larger samples is required to confirm these findings and demonstrate if LF-rTMS and HF-rTMS are similarly efficacious, or if LF-rTMS is more efficacious than HF-rTMS. Aside from the comparison of clinical effects, it appears that LF-rTMS is better tolerated i.e. patients reported less headaches. It may also minimize the risk of inducing adverse events like seizures (Rossi et al., 2009).
Although the vast majority of studies have focused on low frequency stimulation applied to the right and high frequency stimulation applied to the left dorsolateral prefrontal cortex, it is to be noted that in a few studies parameters have varied from these traditional sites. In one of large study in 219 depressed patients, no effects of laterality and frequency were found while all patients showed a reduction in their depression symptoms (Fitzgerald et al., 2010). This may implicate that low frequency stimulation applied to the left may also have antidepressant effects, thus questioning the traditional model of laterality and frequency in depression, with respect to the DLPFC.
Combined HF and LF-rTMS protocols.
These aforementioned studies demonstrate evidence that active HF-rTMS and LF-rTMS are more effective in the treatment of depression as compared to sham. However, HF-rTMS and LF-rTMS are not necessarily incompatible with each other. In recent years, add-on, bilateral -sequential and priming protocols have been postulated and investigated.
Add-on protocols concern the combination of one protocol with another protocol e.g. when patients do not respond to LF-rTMS after several sessions, they can proceed to HF-rTMS treatment. In the aforementioned study by Fitzgerald et al. (2006) in which LF-rTMS was investigated, non-responders to the low frequency protocol were subsequently treated with HF-rTMS. A subset of these LF-rTMS non-responders did respond to HF-rTMS. Hence, it is likely that different protocols act through different mechanisms and that different patient groups are receptive to different approaches. It could also be argued that subjects in the add-on protocol received more sessions, and possibly needed longer to respond to treatment. Thus, the full extent of the increase in response rate might not solely be attributed to the change in stimulation frequency.
A second variant is the sequential stimulation protocol in which within one session both HF-rTMS and LF-rTMS protocols are applied. Overall no superiority has been found of sequential bilateral rTMS (Fitzgerald, Huntsman, Gunewardene, Kulkarni, & Daskalakis, 2006), except for Blumberger and colleagues (Blumberger et al., 2012) who found added value of this approach, albeit their unilateral HF rTMS did not differ from sham. Finally, in a recent study by Pallanti et al. (Pallanti, Bernardi, Rollo, Antonini, & Quercioli, 2010) found that that a ‘simple’ unilateral protocol was more effective compared to sequential bilateral rTMS. Nevertheless, it remains relevant to further explore combination protocols and compare them to traditional unilateral protocols, also looking at this option as an escalation option to increase the ‘dose’, i.e. when patients do not respond well enough in 10-15 sessions unilateral rTMS. Since number of stimuli were kept the same at 420 stimuli for both unilateral and bilateral protocols (2010), adding the contralateral hemisphere and thereby doubling the number of pulses, could still have added value by increasing number of pulses, which has in our experience been found to sometimes provide benefit (unpublished observation).
Real-life outcomes and durability
Several large open label studies have addressed the long-term effects of rTMS. In a large multicenter study with 307 treatment resistant MDD patients (failure of on average 2.5 anti-depressant treatments of adequate dose and without satisfactory benefit), Carpenter and colleagues (2012) reported clinician-assessed response rates (CGI-S) of 58.0% and remission of 37.1%. Patient-reported response rate ranged from 56.4 to 41.5% and remission rate ranged from 28.7 to 26.5%. The authors note that these outcomes demonstrated response and adherence rates similar to research populations. Another large open label study in 1132 patients demonstrated similar effects to the Carpenter et al. (2012) study with 46% response and 31% remission rates (Fitzgerald, Hoy, Anderson, & Daskalakis, 2016). In an extension of the Carpenter et al. (2012) study, good long-term effects were observed (Dunner et al., 2014). The majority of the patients (62.5 %) continued to meet response criteria at a 12 month follow-up. Similar results were reported by Donse and colleagues (2018) who conducted a combined rTMS and psychotherapy study while also investigating long term effects in 196 patients with TRD. Combining rTMS and psychotherapy resulted in a 66% response and a 56% remission rate at the end of treatment with 60% sustained remission at a six-month follow-up visit. The authors noted that, interestingly, early symptom improvement (at session 10) was highly predictive of response, and may therefore be used to guide rTMS plus psychotherapy continuation, or escalation to sequential HF and LF rTMS.
As such, it seems that within a naturalistic setting, rTMS can be considered an effective treatment similar to that found in a research setting. Additionally, in combination with psychotherapy, response and remission rates may have the potential to increase further and sustain durable effects. As can be seen in figure 3, a treatment resistant population respond to rTMS alone (monotherapy) similarly to an anti-depressant medication (monotherapy) in populations receiving medication or psychotherapy as a first line treatment; and, when combined with psychotherapy, rTMS patients respond similarly to a combined medication/psychotherapy approach. These findings suggest a considerable promise for this hard to treat population following many prior failed attempts at depressive symptom reduction.
Figure 3: Response and remission rates of various antidepressant treatments from left to right, Psychotherapy monotherapy (Cuijpers et al., 2014), Psychotherapy and antidepressants (Keller et al., 2000), Antidepressants as first line, after one, two and three treatment failures from the STAR*D trial (Rush et al., 2006), rTMS monotherapy (Carpenter et al., 2012) and rTMS combined with psychotherapy (Donse et al., 2018). Note the relative increase in response and remission rates for rTMS, especially for patients that have had two or three prior treatment failures, which is the typical population rTMS treatment focusses on (TRD).
Stimulation location
The dorsolateral prefrontal cortex (DLPFC) has been the primary area of interest for stimulation (see Figure 4). The motivation behind choosing this brain area stems from various imaging studies that indicated depression is associated with regional brain dysfunction in, among other regions, the DLPFC (Cummings 1993). Other researchers have not only proposed an ‘underactivated’ L-DLPFC, but suggested an imbalance between frontal regions. For example, the ‘frontal asymmetry hypothesis’ of depression states that in depression there is an imbalance in left vs. right frontal brain activation (Henriques & Davidson, 1990). Although a recent meta-analyses failed to demonstrate a difference in frontal alpha asymmetry between depressed and non-depressed groups on the group level (van der Vinne, Vollebregt, van Putten, & Arns, 2017), the DLPFC is still the most commonly used stimulation location in rTMS protocols for depression. In addition, of all brain regions known to be related to the pathophysiology of depression (e.g., prefrontal, cingulate, orbitofrontal and parietal cortical regions) the DLPFC is regarded as most accessible for treatment with rTMS (Wassermann & Lisanby, 2001). On the basis of such previous theories and findings, the supposedly ‘activating’/ HF-rTMS protocols are applied over the left DLPFC and supposedly ‘inhibiting’/LF-rTMS protocols are applied over the right DLPFC. The choice of the stimulation frequency is thus closely linked to the stimulation location.
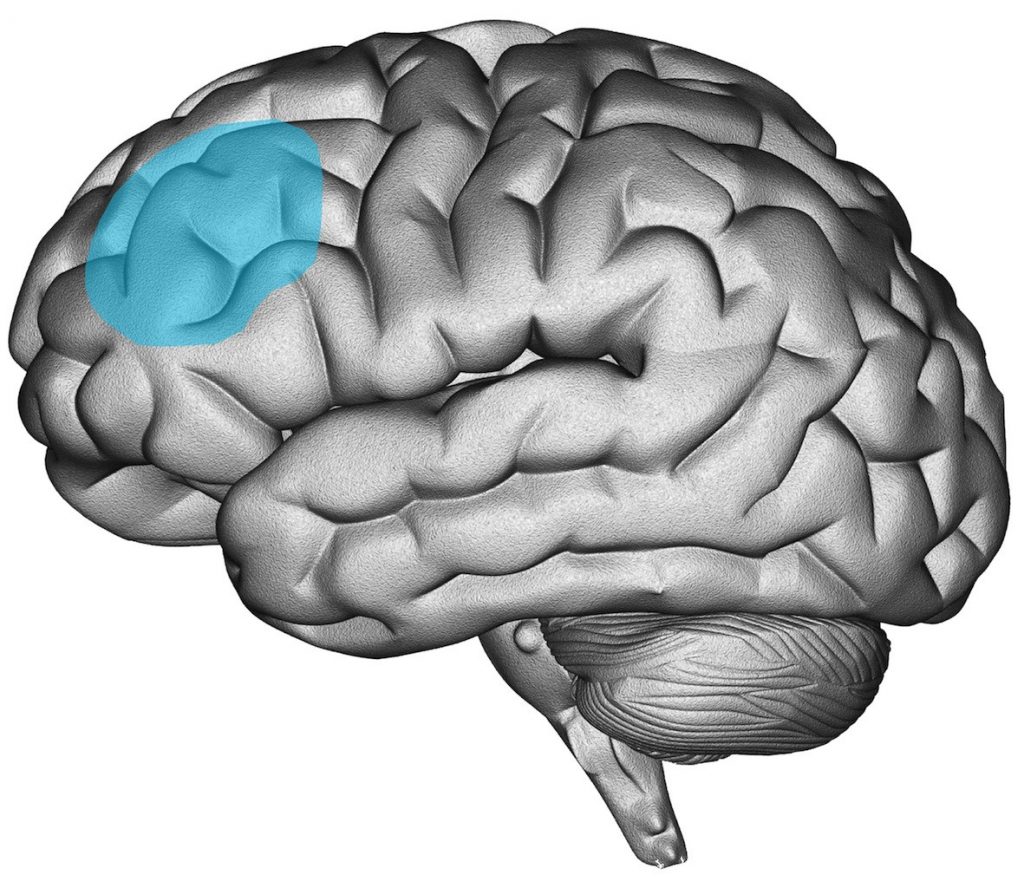
Dlpfc Left
Figure 4: Image of the location of the (left) Dorsolateral Prefrontal Cortex in the brain (Figure used with permission from Research Institute Brainclinics ©)
Historically, most studies localizing the DLPFC have been performed by means of the ‘5cm rule’. The hand area of the primary motor cortex (M1) (which elicits a contralateral motor response of the thumb when stimulated), is taken as the detectable reference point. From there, the coil is moved 5 cm anteriorly, in a sagittal direction. Positioning the coil at that location during treatment is assumed to target the DLPFC. It can be argued that this literal “rule of thumb” has some flaws and may result in inconsistent results between sessions within subjects, and is used less frequently these days. Recently, the Beam-F3 / F4 method has been proposed as a new method (Beam, Borckardt, Reeves, & George, 2009) which does take individual differences in skull size into account and is based on the 10-20 EEG location F3 or F4. Free software to easily apply this method can be found at: http://www.clinicalresearcher.org/software.htm. This method has been shown to lead to an adequate determination, with a minimal discrepancy, compared to MRI neuro – navigated location determination (Mir-Moghtadaei et al., 2015).
For MRI neuronavigation, a technique in which an individual MRI scan is used to determine the exact location of the DLPFC, a single study has found an advantage regarding clinical effectiveness in the treatment of TRD compared to the 5 cm rule (Fitzgerald et al., 2009). However, results from the multi-center OPT-TMS trial in which an individual adjustment could be made based on an individual MRI scan, showed no improved clinical effect of this MRI-based adaptation (Johnson et al., 2013), and there are no comparative studies that compared the effectiveness of MRI-navigated rTMS with the Beam-F3 / F4 method.
Despite the fact that the majority of the studies target the DLPFC, some authors have argued that it has never been experimentally proven that the DLPFC is the most effective target for rTMS treatment of depression. In addition, the pathophysiology of depression is certainly not limited to the DLPFC (Drevets, Price, & Furey, 2008). Therefore, several other stimulation targets have also been investigated in the treatment of depression. Clinical efficacy in TRD has also been reported after stimulation of the Dorsomedial Prefrontal cortex or Anterior Cingulate Cortex using a double-cone coil (Downar et al., 2014; Kreuzer et al., 2015), the right orbitofrontal cortex using a double cone coil FP2/AF8 (Feffer et al., 2018) and a limited effect in the stimulation of the right parietal cortex (P4: (Schutter 2009)). Although these findings need to be replicated in larger studies, they are encouraging regarding searching for other cortical targets in the treatment of depression with rTMS outside the DLPFC.
Stimulation intensity, trains, sessions and safety
For rTMS to be effective, the magnetic field has to induce currents in the neurons of the cortex. The intensity of the magnetic field that induces this current is referred to as the stimulation intensity. This is usually expressed as a percentage of the motor threshold (MT). The MT is usually determined prior to each session by applying the TMS coil over the ‘thumb’ area of the motor cortex. Single pulses are applied by stepwise variation of the output intensity of the device. The minimal output intensity which yields a motor response (moving of the thumb) in at least half of the applied trials is determined to be the MT. So if the intensity of a TMS protocol is 100% MT, then it is the same as the output intensity of the device which was determined to be MT. All other intensity values are reflected as a percentage of this MT, e.g. if the MT is at an output intensity of the device of 60%, then an intensity of 110% MT means that the output intensity is 66%. Although this determination of stimulation intensity may seem arbitrary, it takes individual differences in motor cortex excitability (and therefore excitability of other brain regions) into account. This contributes to a safer administration of TMS pulses to an individual. In depression protocols reported to date, the lowest stimulation intensity used was 80% MT (George et al., 1995) and the maximal intensity used was 120% MT (O’Reardon et al., 2007; Rumi et al., 2005). The majority of the depression protocols to date use stimulation intensities of 110%-120% MT.
In most rTMS protocols the stimulation is delivered in pulse trains (see Figure 2). That is, pulses are delivered in trains and are separated by certain time intervals: the inter train interval (ITI). This is done for two reasons. First, the effect of TMS pulses is cumulative in the brain (Hallett 2007; Ridding & Rothwell, 2007; Rossi & Rossini, 2004), and this summation causes an increase of the likelihood of the induction of a seizure. This possible side effect of rTMS – the occurrence of an epileptic seizure – is minimized when adhering to the published safety guidelines (Rossi et al., 2009; Wassermann 1998) that have been composed based on reported incidents. These guidelines concern stimulation parameters (such as frequency of stimulation (Hz), intensity of stimulation (as a percentage of the ‘motor threshold’ (MT), and duration of a stimulation train), as well as other risk factors which should be taken into account (such as sleep deprivation, acute withdrawal of benzodiazepines etc.). Since the publication of these and previous safety guidelines (Rossi et al., 2009; Wassermann, 1998), only few reported incidents of epileptic seizures are known. Currently, the risk of a seizure due to rTMS, according to the literature from research on a global level, is 0.007% (20 seizures / 300,000 sessions) and 0.003% (7 seizures / 250,000 sessions, 0.1% / patients) in clinical practice. This means that the risk of a seizure is comparable or lower than with antidepressants (George, Taylor, & Short, 2013).
Secondly, the repetitive release of strong electrical pulses causes heating of the electronics of the TMS device. The ITI between trains allows the device to partially cool down. Due to safety reasons for the subject and protection of the device, all devices are manufactured to automatically turn off as soon as a certain heat-limit has been reached. Newer TMS devices are designed with better cooling systems (e.g. air or fluid cooled coils), which reduce the likelihood of overheating. However, the overheating of the device is still possible when multiple sessions are performed within a short period, or if a highly demanding (e.g. high rate of pulse delivery) protocol is performed. Train durations in HF-rTMS protocols are usually between 2 and 10 seconds with an ITI between 20-60 seconds. In LF-rTMS protocols often, continuous stimulation is used.
In studies performed thus far, the number of sessions applied has been highly variable, ranging from 5 sessions (Manes et al., 2001; Miniussi et al., 2005) to up to or greater than 30 sessions (Fitzgerald et al., 2006; O’Reardon et al., 2007). Based on more recent studies, a general trend towards a greater number of sessions (>10) are associated with continuing improvement in depression scores. Schutter (Schutter 2009) suggested that similar to antidepressant medication, rTMS treatment may involve a delayed therapeutic onset. Investigation of the number of sessions optimally required is important for gaining information about the temporal course of the antidepressant effect.
Over the years of rTMS research efficacy has increased in line with increased dosage, which can be expressed as number of pulses applied during a treatment course. Initially, George and colleagues (1995), applied a total of only 5000 pulses. This was doubled in 1996 by Pasqual-Leone and colleagues, escalating to 10 000 pulses. By 2007, O’Reardon and colleagues had increased to 90 000 pulses, which continued to be well tolerated (4.5% dropout) and efficacy still rising. At this point in time, it seems additional pulses have ceased to have a cumulative effect and by this point a patient has either responded, or has not. But further investigation is warranted.
The variety of protocols discussed above indicate that rTMS is an active field of research. Treatment outcome has been shown to vary with protocols, but some protocols have proven their efficacy. However, it has been argued that it is unlikely that the current combinations of stimulation parameters potentiate optimum clinical effects. It is likely that there is room for improvement, and studies directly addressing the question of optimal stimulation parameters are urgently required. This statement is further supported by the finding that early rTMS depression protocols have shown less favorable results compared to relatively newer, more promising protocols (Gross, Nakamura, Pascual-Leone, & Fregni, 2007). Increasing knowledge about the mechanisms underlying treatment efficacy – the topic of the next section – may result in new protocols with closer to optimal treatment effects.
Mechanisms of rTMS treatment in depression
Levels of evidence for the treatment of depression via rTMS have accumulated to a point where HF-rTMS on left-DLPFC has received Level A status (‘definite efficacy’, Lefaucheur et al. 2014). Only HF-rTMS of M1 contralateral to pain side for the treatment of neuropathic pain has also achieved this status. Level B status (‘probable efficacy’) has been achieved for treatment of depression using LF-rTMS on right-DLPFC and is ‘probably’ additive to anti-depressant medication. Level B evidence has also been achieved for LF-rTMS of M1 contralateral to pain side; antidepressant effect of HF-rTMS of the left DLPFC in PD patients; LF-rTMS of the contra-lesional motor cortex in chronic motor stroke; and, HF-rTMS of the left DLPFC for negative symptoms of schizophrenia (Lefaucheur et al., 2014). Regardless of these evidence levels, the neural mechanisms underlying brain modulation via rTMS remain elusive.
Depression network
Since the rise of neuromodulation treatments for depression such as rTMS and Deep Brain Stimulation (DBS) (Fox, Buckner, White, Greicius, & Pascual-Leone, 2012; Liston et al., 2014), new hubs in the depression network have been identified. These new treatments directly targeted DLPFC (George et al., 2010; O’Reardon et al., 2007), the dorsomedial prefrontal cortex (DMPFC) (Downar & Daskalakis, 2013; Downar et al., 2014) and the subgenual cingulate cortex (sgACC) (Mayberg et al., 2005) and have shown that direct stimulation of these regions is associated with clinical improvement. Recent insights into how these neuromodulation treatments work suggest network connectivity changes within a DLPFC-DMPFC-ACC network to mediate antidepressant response (Fox et al., 2012; Liston et al., 2014), and are also possibly implicated in pharmacological treatments. Moreover, new targets are still identified, such as the orbitofrontal cortex (Frodl et al., 2010).
The sgACC and sections of the DMPFC are components of the default mode network while the DLPFC is partly implicated in the central executive network (CEN). A deficit in switching between the DMN and CEN is well known in depression (Liston et al., 2014; Sridharan, Levitin, & Menon, 2008) and is considered to be one of the main reasons behind cognitive dysfunction in depression. The DLPFC has been described to be hypoactive in depression (Korgaonkar, Grieve, Etkin, Koslow, & Williams, 2012), and an increase in fMRI activity of this structure is associated with treatment response (Fitzgerald et al., 2006; Koenigs & Grafman, 2009). This hypoactivation correlates to the amount of grey matter volume reduction (Grieve, Korgaonkar, Koslow, Gordon, & Williams, 2013). Contrary to the DLPFC, the sgACC has been described to be hyperactive in depression, along with hyperconnectivity to other parts of the DMN observed with PET scans and with fMRI (Liston et al., 2014; Mayberg et al., 2005), and a decrease in activity of the sgACC is associated with antidepressant response (Koenigs & Grafman, 2009; Mayberg et al., 2005), also decreased grey matter volume in MDD has been reported in the left anterior cingulate cortex (Drevets et al., 1997; Grieve et al., 2013). The DMPFC, or dorsal nexus, is a core region to multiple networks, including the DMN, CEN and salience network (SN), with increased fMRI connectivity to all three networks in depression (Sheline, Price, Yan, & Mintun, 2010). The DMPFC has been observed to be abnormally activated during positive and negative affect processing in MDD, which normalizes after successful treatment (Bermpohl et al., 2009; Dunlop, Hanlon, & Downar, 2015; Mayberg et al., 1999).
As rTMS is limited to cortical surfaces, it is hypothesized that DLPFC-rTMS (and DMPFC-rTMS) might exert its antidepressant effect via trans-synaptic connectivity to deeper regions, such as the sgACC (Fox et al., 2014; Fox et al., 2012; George et al., 1997, 1995; Padberg & George, 2009). Fox et. al., (2012) demonstrated a negative correlation between the sgACC and the DLPFC which is hypothesized to be associated to the antidepressant mechanism of rTMS (Fox et al., 2012). The higher the negative correlation in activity between the two areas was, the better a patient responded to rTMS (2012). In addition, DBS in the sgACC which suppresses activity, results in an up regulation of the activity in the DLPFC (Mayberg et al., 2005). Thus, irrespective of the direction and causality, there is an intricate interplay between the sgACC and DLPFC, and this interplay is related to MDD symptom (improvement). This interplay has also been investigated during antidepressant treatment, and it was shown that electroencephalogram (EEG) linear-lagged connectivity between the DLPFC and the sgACC changed for responders during treatment, however this was only observed in males (Iseger et al., 2017). Multiple studies have described a normalization of the existing hyper-connectivity (Koenigs & Grafman, 2009; Liston et al., 2014; Mayberg et al., 2005) after treatment. However, some studies still report differences in ACC or DLPFC connectivity after treatment, compared to healthy controls. Therefore it was hypothesized that connectivity tends to normalize or resemble the connectivity pattern of healthy controls, but could still be incomplete (Liston et al., 2014).
These hubs in depression are linked to the limbic system, such as the amygdala and hippocampus but there are convergent findings as to whether metabolic changes and volume reductions associated with depression are present in these structures (Grieve et al., 2013). When investigating white matter trajectories, significant reductions were found in regions associated with the limbic system, but only in the melancholic subtype of depression (Korgaonkar et al., 2010). Moreover, it is believed that this depression network is connected to the heart. This is not surprising because MDD has been strongly associated with a higher risk for cardiovascular disease (Glassman, 2007; Lett et al., 2004; Penninx et al., 2001). Several studies have shown that depression increases the risk for cardiovascular illness from two to fivefold (Horrobin & Bennett, 1999). Moreover, autonomic regulation is already disturbed in depressed patients without heart disease, manifested in an overall higher HR, and lower heart HRV in comparison to healthy controls (Ehrenthal, Herrmann-Lingen, Fey, & Schauenburg, 2010; Licht et al., 2008; Udupa et al., 2007), and is more pronounced in patients with severe MDD (Stein, Talcott, & Walsh, 2000).
The connection to the heart is mediated by the vagus nerve, the 10th cranial nerve. The vagus nerve is part of the parasympathetic nervous system and, besides regulating heart rate, is also involved in other autonomic functioning such as pupil dilation, sweat response, saliva production, blood pressure, inflammation and gastrointestinal function. Of these, gastrointestinal and heart function have been studied most extensively with regards to depression. Heart rate consistently decreases when the vagus nerve is stimulated (Buschman et al., 2006; Lang & Levy, 1989), which is in line with the parasympathetic action of the vagus nerve. Conversely, strong positive correlations were found between cardiac vagal control and the BOLD signal intensity in the sgACC of healthy controls but not in depressed subjects (Lane et al., 2013). Moreover, RR intervals (intervals between ventricular depolarizations) were positively related with VMPFC activity in healthy participants during rest (Ziegler, Dahnke, Yeragani, & Bär, 2009) and patients with ACC damage all showed abnormalities in cardiovascular arousal during cognitive tasks, indicating a blunted autonomic arousal response during stressful situations (Critchley et al., 2003). Few studies have been conducted investigating the effect of neuromodulation on the VMPFC in relation to heart rate, however one older study on monkeys showed electrical stimulation in the pregenual ACC associated with cardiac slowing (DUA & MACLEAN, 1964). The same associations with heart rate control have been found for the DLPFC. A recent meta-analysis demonstrated that both tDCS and rTMS aimed at the DLPFC resulted in reduced heart rate, although rTMS was more effective than tDCS (Makovac, Thayer, & Ottaviani, 2016). These effects were observed both after left and right hemispheric stimulation, although some studies do observe larger heart rate reductions after right DLPFC stimulation.
In summary, the core of the depression network involves the DLPFC and the sgACC, and these regions are interconnected with the limbic system and the heart-brain axis, providing new insights into the pathophysiology of MDD that can be utilized to further optimize treatments such as rTMS, as is explained in more detail below under target engagement regarding Neuro-Cardiac-Guided TMS (NCG TMS).
New Developments
As a new and dynamic field, rTMS is the topic of considerable research and innovative developments are numerous. These developments are of a diverse nature, including technological progress in equipment and software, protocol innovations and optimizations, and advances in the understanding of long-term effects. Some examples are the investigation of theta burst stimulation applicability (the delivery of bursts of 50 Hz pulses usually at a rate of 5 Hz) and new equipment such as the H-coil for deeper brain stimulation.
Progress in protocols
In addition to the ‘traditional’ LF and HF frequency studies, a newly developed theta-burst stimulation (TBS) protocol has been proposed; referred to as ‘patterned TMS’. This has been put forward as a technique that could have important implications for the treatment of conditions such as epilepsy, depression and Parkinson’s Disease (Paulus 2005). TBS usually involves short bursts of 50 Hz rTMS applied at a rate of 5Hz (hence the name theta burst stimulation). In fact there are two frequencies within one train of stimuli; the inter burst frequency of 50 Hz (e.g. 3 pulses at a rate of 50 Hz) and the frequency of delivery of the number of bursts within one second which is at a rate of 5 per second (5 Hz). TBS can be applied as either a continuous (cTBS), or intermittent (iTBS) train (Huang, Edwards, Rounis, Bhatia, & Rothwell, 2005). See Figure 5 for an illustration of both types of TBS protocols.
Figure 5: examples of the two most common TBS protocols: continuous TBS (first trace) and intermittent TBS (second trace). Figure taken and adapted from Rossi et al. (2009
Until recently, the rapid delivery of pulses required in a TBS protocol was not possible due to technical limitation of older stimulators. TBS has therefore only been investigated since 2005. In the years after its introduction, it has been shown that TBS induces changes in cortical excitability that may last longer than with traditional TMS protocols (Huang et al., 2009; Ishikawa et al., 2007). In regard to the observation of the more sustained effect, Chistyakov and colleagues (2010) suggested that TBS might be more effective than traditional HF and LF-rTMS in the treatment of depression. However, recent work has demonstrated that TBS protocols seem to be equally effective in the treatment of depression, but may be more efficient as the session length is shorter (Bakker et al., 2014).
Technical progress
In order to further improve efficacy of rTMS protocols, a new type of coil ‘the H-coil’ (Brainsway) was developed. The traditionally used coils (figure of eight/circular coils) are thought to penetrate underlying brain tissue only to a depth of 1.5-2cm (Zangen, Roth, Voller, & Hallett, 2005). H-coils, on the other hand, are capable of stimulating deeper brain regions of 4-6 cm (2005), albeit others have argued this coil results in more non-focal, but not deeper stimulation (Fadini et al., 2009).
The H-coil was thus developed to target deeper cortical regions. In 2013 the U.S. Food and Drug Administration (FDA) approved the Deep TMS device (using the H-coil) for the treatment of depression in patients who had failed to respond to antidepressant medications in their current episode of depression. The study that resulted in this FDA approval was published in 2015 by Levkovitz and colleagues (Levkovitz et al., 2015) who conducted a double-blind randomized controlled multicenter study evaluating the efficacy and safety of dTMS in MDD. 212 MDD outpatients, aged 22–68 years, who had either failed one to four antidepressant trials or not tolerated at least two antidepressant treatments during the current episode were randomly assigned to active or sham dTMS. Twenty sessions of dTMS (18 Hz over the prefrontal cortex) were applied during 4 weeks acutely, and then bi-weekly for 12 weeks. Response and remission rates were higher in the dTMS than in the sham group (HDRS – response: 38.4 vs. 21.4%; remission: 32.6 vs. 14.6%). These differences between active and sham treatment were stable during the 12-week maintenance phase.
For the time being, randomized head-to-head comparisons of effectiveness on reduction of depressive symptoms between the H-coil and regular figure-of-eight coils have not been published yet. However, the H-coil is a prime example of further technological advancements which may lead to increased efficacy in time.
Optimizing treatment
A better understanding of the neurophysiological and clinical features of depressed patients who respond to rTMS, together with more clarity on the neurobiological mechanisms of the induced effect of rTMS treatment in depression as described above in depression network, will contribute to the development of more effective forms of rTMS. The identification of patients’ characteristics (clinical, physiological or parametric variables) is referred to as personalized medicine. Demographic and clinical features associated with less favorable treatment outcome are older age (Brakemeier, Luborzewski, Danker-Hopfe, Kathmann, & Bajbouj, 2007; Fregni et al., 2006) and higher therapy resistance (Brakemeier et al., 2007; Brakemeier et al., 2008; Fregni et al., 2006). However, this has not been confirmed by all studies in this area (Fitzgerald et al., 2006).
A recent systematic review on predictors for rTMS response concluded that due to methodological variability generalizability of results was limited, but some indications were found for baseline frontal lobe blood flow and presence of some polymorphisms (5-hydroxytryptamine-1a gene, the LL genotype of the serotonin transporter linked polymorphic region (5-HTTLPR) gene, and Val/Val homozygotes of the brain-derived neurotrophic factor (BDNF)) (Silverstein et al., 2015). While EEG measures have been relatively well studied in the prediction of treatment response to antidepressant medication (Bruder et al., 2008; Cook et al., 1999; Spronk, Arns, Barnett, Cooper, & Gordon, 2011), their potential in predicting response to rTMS treatment is generally considered limited but promising, albeit there is a large need for replicated findings (see (Arns, Drinkenburg, Fitzgerald, & Kenemans, 2012; Krepel et al., 2018)) as an example of non-replicated EEG predictors in 2 relatively large samples). Thus, current predictors are still rather limited and require further replication (in larger samples) before their use in clinical practice can be recommended.
Target engagement
Target engagement comprises the use of a direct functional outcome measure as a validation for targeting the correct TMS location, whereby it can be demonstrated that the right area is activated, either directly or trans-synaptically. In the same way as the motor cortex is identified by thumb movement as a demonstration of primary motor cortex activation, such functional outcome measures are thus far lacking for the prefrontal cortex and DLPFC. One proposed method is by extracting connectivity patterns to frontal areas using the sgACC as a seed region (Fox et al., 2012; Fox, Liu, & Pascual-Leone, 2013) . Other studies hypothesize that the DLPFC could be more accurately targeted with the aid of heart rate, so called Neuro-Cardiac-Guided TMS (Heart-Brain Coupling) (Iseger, Padberg, Kenemans, Gevirtz, & Arns, 2017). As mentioned earlier, the depression network and the brain-heart axis are interconnected and stimulation of the DLPFC was shown to reduce heart rate (Makovac et al., 2016). The parasympathetic effect on the heart is short-lived; stimulation of the vagus nerve therefore usually results in an immediate response of the heart, typically occurring within the cardiac cycle in which the stimulation occurred, with a peak in heart rate deceleration within 5 seconds (Buschman et al., 2006). The return to a normal HR is very quick after the activity of the vagus nerve is reduced (Shaffer, McCraty, & Zerr, 2014). In a recent study, it was shown that stimulating different prefrontal scalp locations led to different effects on heart rate, and that stimulation of the F4 and F3 locations (10-20 system) resulted in the most significant heart rate decelerations, whereas this was not found for central sites overlying the primary motor cortex (Iseger et al., 2017). Individual variation was also found, however (i.e. for some individuals the most profound heart rate deceleration was found for slightly more posterior sites e.g. FC3, FC4 relative to F3 and F4), indicating that the Heart-Brain Coupling method could be used to individualize the correct stimulation target, under the assumption that trans-synaptic activation of the sgACC indeed activates the whole DLPFC-sgACC-Vagal nerve pathway that is involved in MDD. However, it has yet to be established how this correlates with treatment outcome and if such targeting methods result in increased clinical efficacy.
To summarize, rTMS has gained much empirical support, both from controlled as well as large open-label studies demonstrating clinical efficacy in the treatment of TRD. Many developments are currently ongoing to further optimize treatments such as new stimulation protocols, new coil designs, predictors for treatment response and methods of target engagement, likely resulting in further improved efficacy for the future.
References
- Arns, M. (2010). Historical archives: The beginning. Journal of Neurotherapy, 14(4), 291-292.
- Arns, M., Drinkenburg, P., Fitzgerald, P., & Kenemans, L. (2012). Neurophysiological predictors of non-response to rtms in depression. Brain Stimulation, 5(4), 569-576. doi:10.1016/j.brs.2011.12.003
- Avery, D. H., Holtzheimer, P. E., Fawaz, W., Russo, J., Neumaier, J., Dunner, D. L., . . . Roy-Byrne, P. (2006). A controlled study of repetitive transcranial magnetic stimulation in medication-resistant major depression. Biological Psychiatry, 59(2), 187-94. doi:10.1016/j.biopsych.2005.07.003
- Bakker, N., Shahab, S., Giacobbe, P., Blumberger, D. M., Daskalakis, Z. J., Kennedy, S. H., & Downar, J. (2014). RTMS of the dorsomedial prefrontal cortex for major depression: Safety, tolerability, effectiveness, and outcome predictors for 10 hz versus intermittent theta-burst stimulation. Brain Stimulation.doi:10.1016/j.brs.2014.11.002
- Barker, A. T., Jalinous, R., & Freeston, I. L. (1985). Non-invasive magnetic stimulation of human motor cortex. Lancet,1(8437), 1106-7.
- Beam, W., Borckardt, J. J., Reeves, S. T., & George, M. S. (2009). An efficient and accurate new method for locating the F3 position for prefrontal TMS applications. Brain Stimulation, 2(1), 50-54. doi:10.1016/j.brs.2008.09.006
- Berlim, M. T., Van den Eynde, F., & Daskalakis, Z. J. (2013). Efficacy and acceptability of high frequency repetitive transcranial magnetic stimulation (rtms) versus electroconvulsive therapy (ECT) for major depression: A systematic review and meta-analysis of randomized trials. Depression and Anxiety, 30(7), 614-23. doi:10.1002/da.22060
- Bermpohl, F., Walter, M., Sajonz, B., Lücke, C., Hägele, C., Sterzer, P., . . . Northoff, G. (2009). Attentional modulation of emotional stimulus processing in patients with major depression–alterations in prefrontal cortical regions. Neuroscience Letters, 463(2), 108-13. doi:10.1016/j.neulet.2009.07.061
- Bickford, R. G., Guidi, M., Fortesque, P., & Swenson, M. (1987). Magnetic stimulation of human peripheral nerve and brain: Response enhancement by combined magnetoelectrical technique. Neurosurgery,20(1), 110-6.
- Blumberger, D. M., Mulsant, B. H., Fitzgerald, P. B., Rajji, T. K., Ravindran, A. V., Young, L. T., . . . Daskalakis, Z. J. (2012). A randomized double-blind sham-controlled comparison of unilateral and bilateral repetitive transcranial magnetic stimulation for treatment-resistant major depression. The World Journal of Biological Psychiatry : The Official Journal of the World Federation of Societies of Biological Psychiatry, 13(6), 423-35. doi:10.3109/15622975.2011.579163
- Brakemeier, E. -L., Luborzewski, A., Danker-Hopfe, H., Kathmann, N., & Bajbouj, M. (2007). Positive predictors for antidepressive response to prefrontal repetitive transcranial magnetic stimulation (rtms). Journal of Psychiatric Research, 41(5), 395-403. doi:10.1016/j.jpsychires.2006.01.013
- Brakemeier, E. -L., Wilbertz, G., Rodax, S., Danker-Hopfe, H., Zinka, B., Zwanzger, P., . . . Padberg, F. (2008). Patterns of response to repetitive transcranial magnetic stimulation (rtms) in major depression: Replication study in drug-free patients.Journal of Affective Disorders, 108(1-2), 59-70. doi:10.1016/j.jad.2007.09.007
- Bruder, G. E., Sedoruk, J. P., Stewart, J. W., McGrath, P. J., Quitkin, F. M., & Tenke, C. E. (2008). Electroencephalographic alpha measures predict therapeutic response to a selective serotonin reuptake inhibitor antidepressant: Pre- and post-treatment findings. Biological Psychiatry, 63(12), 1171-7. doi:10.1016/j.biopsych.2007.10.009
- Buschman, H. P., Storm, C. J., Duncker, D. J., Verdouw, P. D., van der Aa, H. E., & van der Kemp, P. (2006). Heart rate control via vagus nerve stimulation. Neuromodulation: Technology at the Neural Interface, 9(3), 214-220.
- Carpenter, L. L., Janicak, P. G., Aaronson, S. T., Boyadjis, T., Brock, D. G., Cook, I. A., . . . Demitrack, M. A. (2012). Transcranial magnetic stimulation (TMS) for major depression: A multisite, naturalistic, observational study of acute treatment outcomes in clinical practice. Depression and Anxiety, 29(7), 587-96. doi:10.1002/da.21969
- Cook, I. A., Leuchter, A. F., Witte, E., Abrams, M., Uijtdehaage, S. H., Stubbeman, W., . . . Dunkin, J. J. (1999). Neurophysiologic predictors of treatment response to fluoxetine in major depression. Psychiatry Research, 85(3), 263-73.
- Critchley, H. D., Mathias, C. J., Josephs, O., O’Doherty, J., Zanini, S., Dewar, B. -K., . . . Dolan, R. J. (2003). Human cingulate cortex and autonomic control: Converging neuroimaging and clinical evidence. Brain : A Journal of Neurology, 126(Pt 10), 2139-52. doi:10.1093/brain/awg216
- Cuijpers, P., Karyotaki, E., Weitz, E., Andersson, G., Hollon, S. D., & van Straten, A. (2014). The effects of psychotherapies for major depression in adults on remission, recovery and improvement: A meta-analysis. Journal of Affective Disorders, 159, 118-26. doi:10.1016/j.jad.2014.02.026
- Cummings, J. L. (1993). The neuroanatomy of depression. The Journal of Clinical Psychiatry, 54 Suppl, 14-20.
- Donse, L., Padberg, F., Sack, A. T., Rush, A. J., & Arns, M. (2018). Simultaneous rtms and psychotherapy in major depressive disorder: Clinical outcomes and predictors from a large naturalistic study. Brain Stimulation, 11(2), 337-345. doi:10.1016/j.brs.2017.11.004
- Downar, J., & Daskalakis, Z. J. (2013). New targets for rtms in depression: A review of convergent evidence. Brain Stimulation,6(3), 231-40. doi:10.1016/j.brs.2012.08.006
- Downar, J., Geraci, J., Salomons, T. V., Dunlop, K., Wheeler, S., McAndrews, M. P., . . . Giacobbe, P. (2014). Anhedonia and reward-circuit connectivity distinguish nonresponders from responders to dorsomedial prefrontal repetitive transcranial magnetic stimulation in major depression. Biological Psychiatry, 76(3), 176-85. doi:10.1016/j.biopsych.2013.10.026
- Drevets, W. C., Price, J. L., & Furey, M. L. (2008). Brain structural and functional abnormalities in mood disorders: Implications for neurocircuitry models of depression. Brain Structure & Function, 213(1-2), 93-118. doi:10.1007/s00429-008-0189-x
- Drevets, W. C., Price, J. L., Simpson, J. R., Todd, R. D., Reich, T., Vannier, M., & Raichle, M. E. (1997). Subgenual prefrontal cortex abnormalities in mood disorders.Nature, 386(6627), 824-827.
- DUA, S., & MACLEAN, P. D. (1964). LOCALIZATION FOR PENILE ERECTION IN MEDIAL FRONTAL LOBE. The American Journal of Physiology,207, 1425-34.
- Dunlop, K., Hanlon, C. A., & Downar, J. (2015). Noninvasive brain stimulation treatments for addiction and major depression. Annals of the New York Academy of Sciences.
- Dunner, D. L., Aaronson, S. T., Sackeim, H. A., Janicak, P. G., Carpenter, L. L., Boyadjis, T., . . . Demitrack, M. A. (2014). A multisite, naturalistic, observational study of transcranial magnetic stimulation for patients with pharmacoresistant major depressive disorder: Durability of benefit over a 1-year follow-up period. The Journal of Clinical Psychiatry, 75(12), 1394-401. doi:10.4088/JCP.13m08977
- Ehrenthal, J. C., Herrmann-Lingen, C., Fey, M., & Schauenburg, H. (2010). Altered cardiovascular adaptability in depressed patients without heart disease. The World Journal of Biological Psychiatry : The Official Journal of the World Federation of Societies of Biological Psychiatry, 11(3), 586-93. doi:10.3109/15622970903397714
- Eranti, S., Mogg, A., Pluck, G., Landau, S., Purvis, R., Brown, R. G., . . . McLoughlin, D. M. (2007). A randomized, controlled trial with 6-month follow-up of repetitive transcranial magnetic stimulation and electroconvulsive therapy for severe depression. The American Journal of Psychiatry, 164(1), 73-81. doi:10.1176/appi.ajp.164.1.73
- Fadini, T., Matthäus, L., Rothkegel, H., Sommer, M., Tergau, F., Schweikard, A., . . . Nitsche, M. A. (2009). H-coil: Induced electric field properties and input/output curves on healthy volunteers, comparison with a standard figure-of-eight coil. Clinical Neurophysiology : Official Journal of the International Federation of Clinical Neurophysiology, 120(6), 1174-82. doi:10.1016/j.clinph.2009.02.176
- Feffer, K., Fettes, P., Giacobbe, P., Daskalakis, Z. J., Blumberger, D. M., & Downar, J. (2018). 1Hz rtms of the right orbitofrontal cortex for major depression: Safety, tolerability and clinical outcomes. European Neuropsychopharmacology : The Journal of the European College of Neuropsychopharmacology, 28(1), 109-117. doi:10.1016/j.euroneuro.2017.11.011
- Fitzgerald, P. B., Benitez, J., de Castella, A., Daskalakis, Z. J., Brown, T. L., & Kulkarni, J. (2006). A randomized, controlled trial of sequential bilateral repetitive transcranial magnetic stimulation for treatment-resistant depression. The American Journal of Psychiatry, 163(1), 88-94. doi:10.1176/appi.ajp.163.1.88
- Fitzgerald, P. B., Brown, T. L., Marston, N. A. U., Daskalakis, Z. J., de Castella, A., & Kulkarni, J. (2003). Transcranial magnetic stimulation in the treatment of depression: A double-blind, placebo-controlled trial. Archives of General Psychiatry, 60(10), 1002.
- Fitzgerald, P. B., Hoy, K., Daskalakis, Z. J., & Kulkarni, J. (2009). A randomized trial of the anti-depressant effects of low- and high-frequency transcranial magnetic stimulation in treatment-resistant depression. Depression and Anxiety, 26(3), 229-34. doi:10.1002/da.20454
- Fitzgerald, P. B., Hoy, K., Gunewardene, R., Slack, C., Ibrahim, S., Bailey, M., & Daskalakis, Z. J. (2010). A randomized trial of unilateral and bilateral prefrontal cortex transcranial magnetic stimulation in treatment-resistant major depression. Psychological Medicine, 1-10. doi:10.1017/S0033291710001923
- Fitzgerald, P. B., Hoy, K. E., Anderson, R. J., & Daskalakis, Z. J. (2016). A study of the pattern of response to rtms in depression. Depression and Anxiety, 33(8), 746-753. doi:10.1002/da.22503
- Fitzgerald, P. B., Huntsman, S., Gunewardene, R., Kulkarni, J., & Daskalakis, Z. J. (2006). A randomized trial of low-frequency right-prefrontal-cortex transcranial magnetic stimulation as augmentation in treatment-resistant major depression. The International Journal of Neuropsychopharmacology / Official Scientific Journal of the Collegium Internationale Neuropsychopharmacologicum (CINP), 9(6), 655-66. doi:10.1017/S1461145706007176
- Fitzgerald, P. B., Oxley, T. J., Laird, A. R., Kulkarni, J., Egan, G. F., & Daskalakis, Z. J. (2006). An analysis of functional neuroimaging studies of dorsolateral prefrontal cortical activity in depression. Psychiatry Research, 148(1), 33-45. doi:10.1016/j.pscychresns.2006.04.006
- Fox, M. D., Buckner, R. L., Liu, H., Chakravarty, M. M., Lozano, A. M., & Pascual-Leone, A. (2014). Resting-state networks link invasive and noninvasive brain stimulation across diverse psychiatric and neurological diseases. Proceedings of the National Academy of Sciences of the United States of America, 111(41), E4367-E4375. doi:10.1073/pnas.1405003111
- Fox, M. D., Buckner, R. L., White, M. P., Greicius, M. D., & Pascual-Leone, A. (2012). Efficacy of transcranial magnetic stimulation targets for depression is related to intrinsic functional connectivity with the subgenual cingulate. Biological Psychiatry.doi:10.1016/j.biopsych.2012.04.028
- Fox, M. D., Liu, H., & Pascual-Leone, A. (2013). Identification of reproducible individualized targets for treatment of depression with TMS based on intrinsic connectivity. NeuroImage, 66, 151-160. doi:10.1016/j.neuroimage.2012.10.082
- Fregni, F., Marcolin, M. A., Myczkowski, M., Amiaz, R., Hasey, G., Rumi, D. O., . . . Pascual-Leone, A. (2006). Predictors of antidepressant response in clinical trials of transcranial magnetic stimulation. The International Journal of Neuropsychopharmacology / Official Scientific Journal of the Collegium Internationale Neuropsychopharmacologicum (CINP), 9(6), 641-54. doi:10.1017/S1461145705006280
- Frodl, T., Bokde, A. L. W., Scheuerecker, J., Lisiecka, D., Schoepf, V., Hampel, H., . . . Meisenzahl, E. (2010). Functional connectivity bias of the orbitofrontal cortex in drug-free patients with major depression. Biological Psychiatry, 67(2), 161-7. doi:10.1016/j.biopsych.2009.08.022
- Garcia-Toro, M., Pascual-Leone, A., Romera, M., Gonzalez, A., Mico, J., Ibarra, O., . . . Tormos, J. M. (2001).British medical journal(Vol. 71, pp. 546-548). Journal of Neurology, Neurosurgery, and Psychiatry.
- George, M. S., Lisanby, S. H., Avery, D., McDonald, W. M., Durkalski, V., Pavlicova, M., . . . Sackeim, H. A. (2010). Daily left prefrontal transcranial magnetic stimulation therapy for major depressive disorder: A sham-controlled randomized trial. Archives of General Psychiatry,67(5), 507-16. doi:10.1001/archgenpsychiatry.2010.46
- George, M. S., Taylor, J. J., & Short, E. B. (2013). The expanding evidence base for rtms treatment of depression. Current Opinion in Psychiatry, 26(1), 13-8. doi:10.1097/YCO.0b013e32835ab46d
- George, M. S., Wassermann, E. M., Kimbrell, T. A., Little, J. T., Williams, W. E., Danielson, A. L., . . . Post, R. M. (1997). Mood improvement following daily left prefrontal repetitive transcranial magnetic stimulation in patients with depression: A placebo-controlled crossover trial. The American Journal of Psychiatry, 154(12), 1752-6.
- George, M. S., Wassermann, E. M., Williams, W. A., Callahan, A., Ketter, T. A., Basser, P., . . . Post, R. M. (1995). Daily repetitive transcranial magnetic stimulation (rtms) improves mood in depression. Neuroreport, 6(14), 1853-6.
- Grieve, S. M., Korgaonkar, M. S., Koslow, S. H., Gordon, E., & Williams, L. M. (2013). Widespread reductions in gray matter volume in depression. NeuroImage. Clinical, 3, 332-9. doi:10.1016/j.nicl.2013.08.016
- Gross, M., Nakamura, L., Pascual-Leone, A., & Fregni, F. (2007). Has repetitive transcranial magnetic stimulation (rtms) treatment for depression improved? A systematic review and meta-analysis comparing the recent vs. The earlier rtms studies. Acta Psychiatrica Scandinavica,116(3), 165-73. doi:10.1111/j.1600-0447.2007.01049.x
- Grunhaus, L., Schreiber, S., Dolberg, O. T., Polak, D., & Dannon, P. N. (2003). A randomized controlled comparison of electroconvulsive therapy and repetitive transcranial magnetic stimulation in severe and resistant nonpsychotic major depression. Biological Psychiatry, 53(4), 324-31.
- Hallett, M. (2007). Transcranial magnetic stimulation: A primer. Neuron, 55(2), 187-99. doi:10.1016/j.neuron.2007.06.026
- Henriques, J. B., & Davidson, R. J. (1990). Regional brain electrical asymmetries discriminate between previously depressed and healthy control subjects. Journal of Abnormal Psychology, 99(1), 22-31.
- Hoflich G, Kasper S, Hufnagel A, Ruhrmann S, Moller HJ. (1993). Application of transcranial magnetic stimulation in the treatment of drug-resistant major depression: A report of two cases. Application of Transcranial Magnetic Stimulation in the Treatment of Drug-resistant Major Depression: A Report of Two Cases., Hum Psychopharmacol 8, 361-365.
- Huang, Y. -Z., Edwards, M. J., Rounis, E., Bhatia, K. P., & Rothwell, J. C. (2005). Theta burst stimulation of the human motor cortex. Neuron, 45(2), 201-6. doi:10.1016/j.neuron.2004.12.033
- Huang, Y. -Z., Rothwell, J. C., Lu, C. -S., Wang, J., Weng, Y. -H., Lai, S. -C., . . . Chen, R. -S. (2009). The effect of continuous theta burst stimulation over premotor cortex on circuits in primary motor cortex and spinal cord. Clinical Neurophysiology : Official Journal of the International Federation of Clinical Neurophysiology, 120(4), 796-801. doi:10.1016/j.clinph.2009.01.003
- Husain, M. M., Rush, A. J., Fink, M., Knapp, R., Petrides, G., Rummans, T., . . . Kellner, C. H. (2004). Speed of response and remission in major depressive disorder with acute electroconvulsive therapy (ECT): A consortium for research in ECT (CORE) report. The Journal of Clinical Psychiatry, 65(4), 485-91.
- Iseger, T. A., Korgaonkar, M. S., Kenemans, J. L., Grieve, S. M., Baeken, C., Fitzgerald, P. B., & Arns, M. (2017). EEG connectivity between the subgenual anterior cingulate and prefrontal cortices in response to antidepressant medication. European Neuropsychopharmacology : The Journal of the European College of Neuropsychopharmacology.doi:10.1016/j.euroneuro.2017.02.002
- Iseger, T. A., Padberg, F., Kenemans, J. L., Gevirtz, R., & Arns, M. (2017). Neuro-Cardiac-Guided TMS (Heart-Brain Coupling): Probing dlpfc-sgacc-vagus nerve connectivity using heart rate–first results. Brain Stimulation.
- Isenberg, K., Downs, D., Pierce, K., Svarakic, D., Garcia, K., Jarvis, M., . . . Kormos, T. C. (2005). Low frequency rtms stimulation of the right frontal cortex is as effective as high frequency rtms stimulation of the left frontal cortex for antidepressant-free, treatment-resistant depressed patients. Annals of Clinical Psychiatry : Official Journal of the American Academy of Clinical Psychiatrists, 17(3), 153-9.
- Ishikawa, S., Matsunaga, K., Nakanishi, R., Kawahira, K., Murayama, N., Tsuji, S., . . . Rothwell, J. C. (2007). Effect of theta burst stimulation over the human sensorimotor cortex on motor and somatosensory evoked potentials. Clinical Neurophysiology : Official Journal of the International Federation of Clinical Neurophysiology, 118(5), 1033-43. doi:10.1016/j.clinph.2007.02.003
- Januel, D., Dumortier, G., Verdon, C. -M., Stamatiadis, L., Saba, G., Cabaret, W., . . . Fermanian, J. (2006). A double-blind sham controlled study of right prefrontal repetitive transcranial magnetic stimulation (rtms): Therapeutic and cognitive effect in medication free unipolar depression during 4 weeks. Progress in Neuro-psychopharmacology & Biological Psychiatry, 30(1), 126-30. doi:10.1016/j.pnpbp.2005.08.016
- Johnson, K. A., Baig, M., Ramsey, D., Lisanby, S. H., Avery, D., McDonald, W. M., . . . Nahas, Z. (2013). Prefrontal rtms for treating depression: Location and intensity results from the OPT-TMS multi-site clinical trial. Brain Stimulation, 6(2), 108-17. doi:10.1016/j.brs.2012.02.003
- Keller, M. B., McCullough, J. P., Klein, D. N., Arnow, B., Dunner, D. L., Gelenberg, A. J., . . . Zajecka, J. (2000). A comparison of nefazodone, the cognitive behavioral-analysis system of psychotherapy, and their combination for the treatment of chronic depression. The New England Journal of Medicine, 342(20), 1462-70.
- Kirsch, I., Deacon, B. J., Huedo-Medina, T. B., Scoboria, A., Moore, T. J., & Johnson, B. T. (2008). Initial severity and antidepressant benefits: A meta-analysis of data submitted to the food and drug administration. PLoS Medicine, 5(2), e45. doi:10.1371/journal.pmed.0050045
- Klein, E., Kreinin, I., Chistyakov, A., Koren, D., Mecz, L., Marmur, S., . . . Feinsod, M. (1999). Therapeutic efficacy of right prefrontal slow repetitive transcranial magnetic stimulation in major depression: A double-blind controlled study. Archives of General Psychiatry, 56(4), 315-20.
- Koenigs, M., & Grafman, J. (2009). The functional neuroanatomy of depression: Distinct roles for ventromedial and dorsolateral prefrontal cortex. Behavioural Brain Research, 201(2), 239-43. doi:10.1016/j.bbr.2009.03.004
- Korgaonkar, M. S., Grieve, S. M., Etkin, A., Koslow, S. H., & Williams, L. M. (2012). Using standardized fmri protocols to identify patterns of prefrontal circuit dysregulation that are common and specific to cognitive and emotional tasks in major depressive disorder: First wave results from the ispot-d study. Neuropsychopharmacology : Official Publication of the American College of Neuropsychopharmacology.doi:10.1038/npp.2012.252
- Korgaonkar, M. S., Grieve, S. M., Koslow, S. H., Gabrieli, J. D. E., Gordon, E., & Williams, L. M. (2010). Loss of white matter integrity in major depressive disorder: Evidence using tract-based spatial statistical analysis of diffusion tensor imaging. Human Brain Mapping.doi:10.1002/hbm.21178
- Krepel, N., Sack, A. T., Kenemans, J. L., Fitzgerald, P. B., Drinkenburg, W. H., & Arns, M. (2018). Non-replication of neurophysiological predictors of non-response to rtms in depression and neurophysiological data-sharing proposal. Brain Stimulation.doi:10.1016/j.brs.2018.01.032
- Kreuzer, P. M., Schecklmann, M., Lehner, A., Wetter, T. C., Poeppl, T. B., Rupprecht, R., . . . Langguth, B. (2015). The ACDC pilot trial: Targeting the anterior cingulate by double cone coil rtms for the treatment of depression. Brain Stimulation, 8(2), 240-6. doi:10.1016/j.brs.2014.11.014
- Lane, R. D., Weidenbacher, H., Smith, R., Fort, C., Thayer, J. F., & Allen, J. J. B. (2013). Subgenual anterior cingulate cortex activity covariation with cardiac vagal control is altered in depression. Journal of Affective Disorders, 150(2), 565-70. doi:10.1016/j.jad.2013.02.005
- Lang, S. A., & Levy, M. N. (1989). Effects of vagus nerve on heart rate and ventricular contractility in chicken. The American Journal of Physiology, 256(5 Pt 2), H1295-302.
- Lefaucheur, J. -P., André-Obadia, N., Antal, A., Ayache, S. S., Baeken, C., Benninger, D. H., . . . Garcia-Larrea, L. (2014). Evidence-based guidelines on the therapeutic use of repetitive transcranial magnetic stimulation (rtms). Clinical Neurophysiology : Official Journal of the International Federation of Clinical Neurophysiology, 125(11), 2150-2206. doi:10.1016/j.clinph.2014.05.021
- Levkovitz, Y., Isserles, M., Padberg, F., Lisanby, S. H., Bystritsky, A., Xia, G., . . . Zangen, A. (2015). Efficacy and safety of deep transcranial magnetic stimulation for major depression: A prospective multicenter randomized controlled trial. World Psychiatry : Official Journal of the World Psychiatric Association (WPA), 14(1), 64-73. doi:10.1002/wps.20199
- Licht, C. M. M., de Geus, E. J. C., Zitman, F. G., Hoogendijk, W. J. G., van Dyck, R., & Penninx, B. W. J. H. (2008). Association between major depressive disorder and heart rate variability in the netherlands study of depression and anxiety (NESDA). Archives of General Psychiatry, 65(12), 1358-67. doi:10.1001/archpsyc.65.12.1358
- Liston, C., Chen, A. C., Zebley, B. D., Drysdale, A. T., Gordon, R., Leuchter, B., . . . Dubin, M. J. (2014). Default mode network mechanisms of transcranial magnetic stimulation in depression. Biological Psychiatry, 76(7), 517-26. doi:10.1016/j.biopsych.2014.01.023
- Makovac, E., Thayer, J. F., & Ottaviani, C. (2016). A meta-analysis of non-invasive brain stimulation and autonomic functioning: Implications for brain-heart pathways to cardiovascular disease. Neuroscience and Biobehavioral Reviews.doi:10.1016/j.neubiorev.2016.05.001
- Manes, F., Jorge, R., Morcuende, M., Yamada, T., Paradiso, S., & Robinson, R. G. (2001). A controlled study of repetitive transcranial magnetic stimulation as a treatment of depression in the elderly. International Psychogeriatrics / IPA, 13(2), 225-31.
- Mayberg, H. S., Liotti, M., Brannan, S. K., McGinnis, S., Mahurin, R. K., Jerabek, P. A., . . . Fox, P. T. (1999). Reciprocal limbic-cortical function and negative mood: Converging PET findings in depression and normal sadness. The American Journal of Psychiatry, 156(5), 675-82.
- Mayberg, H. S., Lozano, A. M., Voon, V., McNeely, H. E., Seminowicz, D., Hamani, C., . . . Kennedy, S. H. (2005). Deep brain stimulation for treatment-resistant depression. Neuron, 45(5), 651-60. doi:10.1016/j.neuron.2005.02.014
- Miniussi, C., Bonato, C., Bignotti, S., Gazzoli, A., Gennarelli, M., Pasqualetti, P., . . . Rossini, P. M. (2005). Repetitive transcranial magnetic stimulation (rtms) at high and low frequency: An efficacious therapy for major drug-resistant depression? Clinical Neurophysiology : Official Journal of the International Federation of Clinical Neurophysiology,116(5), 1062-71. doi:10.1016/j.clinph.2005.01.002
- Mir-Moghtadaei, Caballero, R., Fried, P., Fox, M. D., Lee, K., Giacobbe, P., . . . Downar, J. (2015). Concordance between beamf3 and mri-neuronavigated target sites for repetitive transcranial magnetic stimulation of the left dorsolateral prefrontal cortex. Brain Stimulation.doi:10.1016/j.brs.2015.05.008
- Mogg, A., Pluck, G., Eranti, S. V., Landau, S., Purvis, R., Brown, R. G., . . . McLoughlin, D. M. (2008). A randomized controlled trial with 4-month follow-up of adjunctive repetitive transcranial magnetic stimulation of the left prefrontal cortex for depression. Psychological Medicine, 38(3), 323-33. doi:10.1017/S0033291707001663
- O’Reardon, J. P., Solvason, H. B., Janicak, P. G., Sampson, S., Isenberg, K. E., Nahas, Z., . . . Sackeim, H. A. (2007). Efficacy and safety of transcranial magnetic stimulation in the acute treatment of major depression: A multisite randomized controlled trial. Biological Psychiatry,62(11), 1208-16. doi:10.1016/j.biopsych.2007.01.018
- Padberg, F., & George, M. S. (2009). Repetitive transcranial magnetic stimulation of the prefrontal cortex in depression. Experimental Neurology, 219(1), 2-13. doi:10.1016/j.expneurol.2009.04.020
- Padberg, F., Zwanzger, P., Thoma, H., Kathmann, N., Haag, C., Greenberg, B. D., . . . Möller, H. J. (1999). Repetitive transcranial magnetic stimulation (rtms) in pharmacotherapy-refractory major depression: Comparative study of fast, slow and sham rtms. Psychiatry Research, 88(3), 163-71.
- Pallanti, S., Bernardi, S., Rollo, A. D., Antonini, S., & Quercioli, L. (2010). Unilateral low frequency versus sequential bilateral repetitive transcranial magnetic stimulation: Is simpler better for treatment of resistant depression? Neuroscience.doi:10.1016/j.neuroscience.2010.01.063
- Pascual-Leone, A., Tarazona, F., Keenan, J., Tormos, J. M., Hamilton, R., & Catala, M. D. (1999). Transcranial magnetic stimulation and neuroplasticity. Neuropsychologia, 37(2), 207-17.
- Paulus, W. (2005). Toward establishing a therapeutic window for rtms by theta burst stimulation. Neuron, 45(2), 181-3. doi:10.1016/j.neuron.2005.01.008
- Paus, T., & Barrett, J. (2004). Transcranial magnetic stimulation (TMS) of the human frontal cortex: Implications for repetitive TMS treatment of depression. Journal of Psychiatry & Neuroscience : JPN, 29(4), 268-79.
- Pridmore, S., Bruno, R., Turnier-Shea, Y., Reid, P., & Rybak, M. (2000). Comparison of unlimited numbers of rapid transcranial magnetic stimulation (rtms) and ECT treatment sessions in major depressive episode. The International Journal of Neuropsychopharmacology / Official Scientific Journal of the Collegium Internationale Neuropsychopharmacologicum (CINP), 3(2), 129-134. doi:10.1017/S1461145700001784
- Ridding, M. C., & Rothwell, J. C. (2007). Is there a future for therapeutic use of transcranial magnetic stimulation? Nature Reviews. Neuroscience, 8(7), 559-67. doi:10.1038/nrn2169
- Rosa, M. A., Gattaz, W. F., Pascual-Leone, A., Fregni, F., Rosa, M. O., Rumi, D. O., . . . Marcolin, M. A. (2006). Comparison of repetitive transcranial magnetic stimulation and electroconvulsive therapy in unipolar non-psychotic refractory depression: A randomized, single-blind study. The International Journal of Neuropsychopharmacology / Official Scientific Journal of the Collegium Internationale Neuropsychopharmacologicum (CINP), 9(6), 667-76. doi:10.1017/S1461145706007127
- Rossi, S., & Rossini, P. M. (2004). TMS in cognitive plasticity and the potential for rehabilitation. Trends in Cognitive Sciences, 8(6), 273-9. doi:10.1016/j.tics.2004.04.012
- Rossi, S., Hallett, M., Rossini, P. M., Pascual-Leone, A., & The Safety of TMS Consensus Group. (2009). Safety, ethical considerations, and application guidelines for the use of transcranial magnetic stimulation in clinical practice and research.Clinical Neurophysiology : Official Journal of the International Federation of Clinical Neurophysiology, 120(12), 2008-39. doi:10.1016/j.clinph.2009.08.016
- Rossini, D., Lucca, A., Zanardi, R., Magri, L., & Smeraldi, E. (2005). Transcranial magnetic stimulation in treatment-resistant depressed patients: A double-blind, placebo-controlled trial. Psychiatry Research, 137(1-2), 1-10. doi:10.1016/j.psychres.2005.06.008
- Rumi, D. O., Gattaz, W. F., Rigonatti, S. P., Rosa, M. A., Fregni, F., Rosa, M. O., . . . Marcolin, M. A. (2005). Transcranial magnetic stimulation accelerates the antidepressant effect of amitriptyline in severe depression: A double-blind placebo-controlled study. Biological Psychiatry, 57(2), 162-6. doi:10.1016/j.biopsych.2004.10.029
- Rush, A. J., Trivedi, M. H., Wisniewski, S. R., Nierenberg, A. A., Stewart, J. W., Warden, D., . . . Fava, M. (2006). Acute and longer-term outcomes in depressed outpatients requiring one or several treatment steps: A STAR*D report. The American Journal of Psychiatry, 163(11), 1905-17. doi:10.1176/appi.ajp.163.11.1905
- Schutter, D. (2009). Transcraniale magnetische stimulatie en cerebrale fysiologische processen bij psychiatrische stoornissen. Tijdschrift Voor Psychiatrie, 51(2), 97-105.
- Schutter, D. J. L. G. (2010). Quantitative review of the efficacy of slow-frequency magnetic brain stimulation in major depressive disorder. Psychological Medicine, 40(11), 1789-1795. doi:10.1017/S003329171000005X
- Shaffer, F., McCraty, R., & Zerr, C. L. (2014). A healthy heart is not a metronome: An integrative review of the heart’s anatomy and heart rate variability. Frontiers in Psychology, 5.
- Sheline, Y. I., Price, J. L., Yan, Z., & Mintun, M. A. (2010). Resting-state functional MRI in depression unmasks increased connectivity between networks via the dorsal nexus. Proceedings of the National Academy of Sciences of the United States of America, 107(24), 11020-5. doi:10.1073/pnas.1000446107
- Silverstein, W. K., Noda, Y., Barr, M. S., Vila-Rodriguez, F., Rajji, T. K., Fitzgerald, P. B., . . . Blumberger, D. M. (2015). Neurobiological predictors of response to dorsolateral prefrontal cortex repetitive transcranial magnetic stimulation in depression: A systematic review. Depression and Anxiety, 32(12), 871-91. doi:10.1002/da.22424
- Spronk, D., Arns, M., Barnett, K., Cooper, N., & Gordon, E. (2011). An investigation of EEG, genetic and cognitive markers of treatment response to antidepressant medication in patients with major depressive disorder: A pilot study. Journal of Affective Disorders, 128(1-2), 41-48. doi:10.1016/j.jad.2010.06.021
- Sridharan, D., Levitin, D. J., & Menon, V. (2008). A critical role for the right fronto-insular cortex in switching between central-executive and default-mode networks. Proceedings of the National Academy of Sciences of the United States of America, 105(34), 12569-74. doi:10.1073/pnas.0800005105
- Stein, J., Talcott, J., & Walsh, V. (2000). Controversy about the visual magnocellular deficit in developmental dyslexics. Trends in Cognitive Sciences, 4(6), 209-211.
- Udupa, K., Sathyaprabha, T. N., Thirthalli, J., Kishore, K. R., Lavekar, G. S., Raju, T. R., & Gangadhar, B. N. (2007). Alteration of cardiac autonomic functions in patients with major depression: A study using heart rate variability measures. Journal of Affective Disorders, 100(1-3), 137-41. doi:10.1016/j.jad.2006.10.007
- van der Vinne, N., Vollebregt, M. A., van Putten, M. J., & Arns, M. (2017). Frontal alpha asymmetry as a diagnostic marker in depression: Fact or fiction? A meta-analysis. NeuroImage: Clinical.
- Wassermann, E. M. (1998). Risk and safety of repetitive transcranial magnetic stimulation: Report and suggested guidelines from the international workshop on the safety of repetitive transcranial magnetic stimulation, june 5-7, 1996. Electroencephalography and Clinical Neurophysiology, 108(1), 1-16.
- Wassermann, E. M., & Lisanby, S. H. (2001). Therapeutic application of repetitive transcranial magnetic stimulation: A review. Clinical Neurophysiology : Official Journal of the International Federation of Clinical Neurophysiology, 112(8), 1367-77.
- Zangen, A., Roth, Y., Voller, B., & Hallett, M. (2005). Transcranial magnetic stimulation of deep brain regions: Evidence for efficacy of the h-coil. Clinical Neurophysiology : Official Journal of the International Federation of Clinical Neurophysiology, 116(4), 775-9. doi:10.1016/j.clinph.2004.11.008
- Ziegler, G., Dahnke, R., Yeragani, V. K., & Bär, K. -J. (2009). The relation of ventromedial prefrontal cortex activity and heart rate fluctuations at rest. European Journal of Neuroscience, 30(11), 2205-2210.